With a combination of our patented arch design providing optimized surface contact and our Surface By Design® Technology encouraging bone cell proliferation, our SPIRA® implants provide the ideal environment for bony ingrowth.
A Future Inspired By The Past
Arches date back to the early Mediterranean civilizations, Mesopotamia, Greece, Persia, and Ancient Italy. However, these civilizations’ arches were mainly reserved for underground architecture and drainage systems to disperse the force from the earth above it. The Romans adopted the Arch from the Etruscans of Tuscany and were the first to use it more widely. Using the Arch allowed the Romans to build longer roads, more significant buildings, and stronger aqueducts. The Arch now proves to be a powerful design feature of SPIRA spine interbody fusion devices.
SPIRA’s proprietary 3D Hierarchal Design, is designed for Stability, Synergy, and Simplicity to drive a fast and reliable interbody fusion.
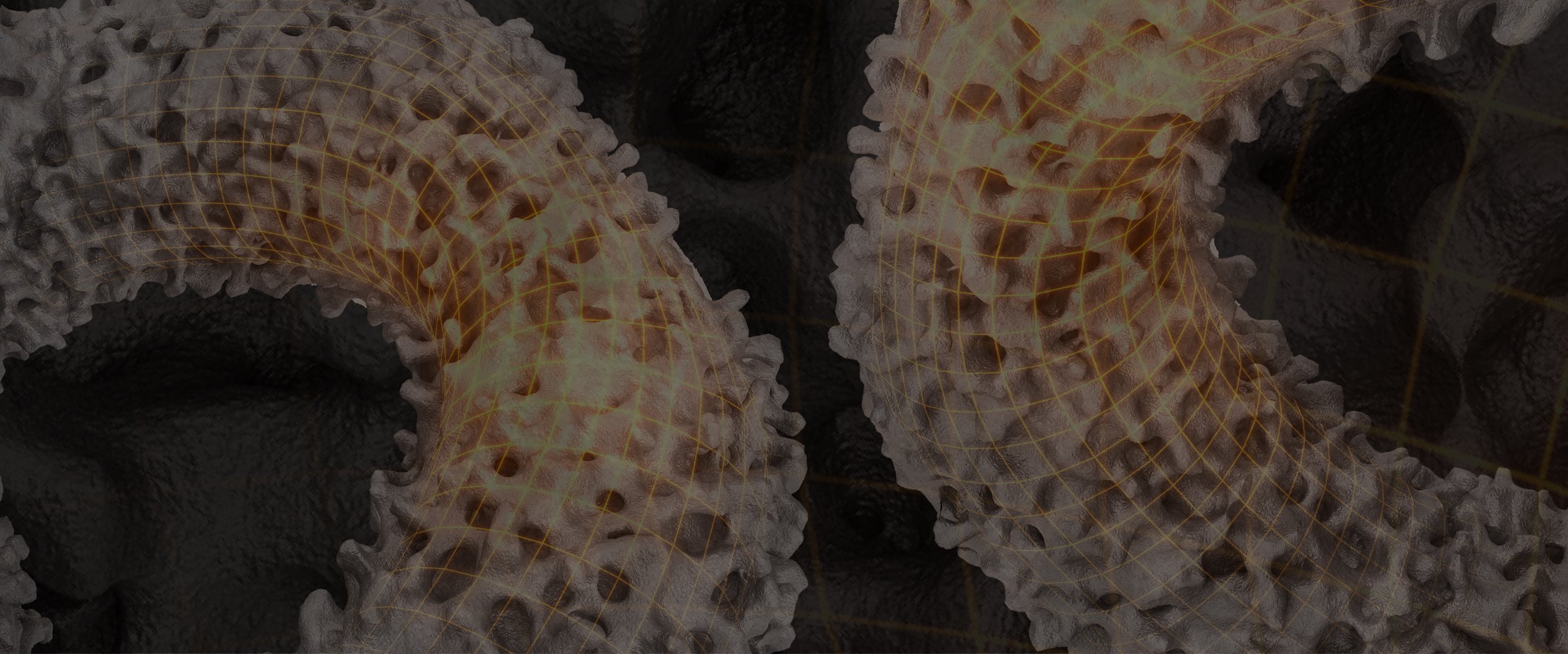
The Power of the Arch
Advantages of the Arch Design
SPIRA’s architecture exploits the advantages of arch design which distributes load to create strength while minimizing titanium volume and allowing maximum space for through-bone growth. SPIRA’s arches are optimized, providing physiologic compressive forces while reducing the tensile forces.
Newly-forming bone grows on to and through the multiple roughened titanium arches to achieve maximum stability and complete endplate-to-endplate arthrodesis.
The arched design enables a distribution of load and stress throughout the cage, which is known from Wolff’s Law¹ to enhance fusion, and protects fusion zones from stress.
3D Printed Titanium
The natural hydrophilicity of titanium has been associated with osseointegration by upregulation of gene expression and protein secretion³. 3D printing has expanded the surface microenvironment increasing the Osteopromotive properties of titanium implants⁴, which greatly reduces the opportunity for biofilm formation by shielding the surface from bacterial contamination⁵.
Allows for a Larger Biologic Footprint
SPIRA Technology interbody cages contain up to approximately 80% porosity and are structurally similar to trabecular bone. As the new bone forms through the cage, it will grow onto and attach to the surfaces of the implant. This is a vital difference from traditional cages that only have bony ongrowth at the endplates.
Subsidence Resistant
The “snowshoe” concept allows for surface area distribution of the loads from initial implantation. This concept of increasing surface area with subsidence allows for decreasing bone stress (load/area) reducing the likelihood of further subsidence. As the healing progresses, the contact surface area continues to increase with further reductions in bone stress and decreasing probability of subsidence.⁶
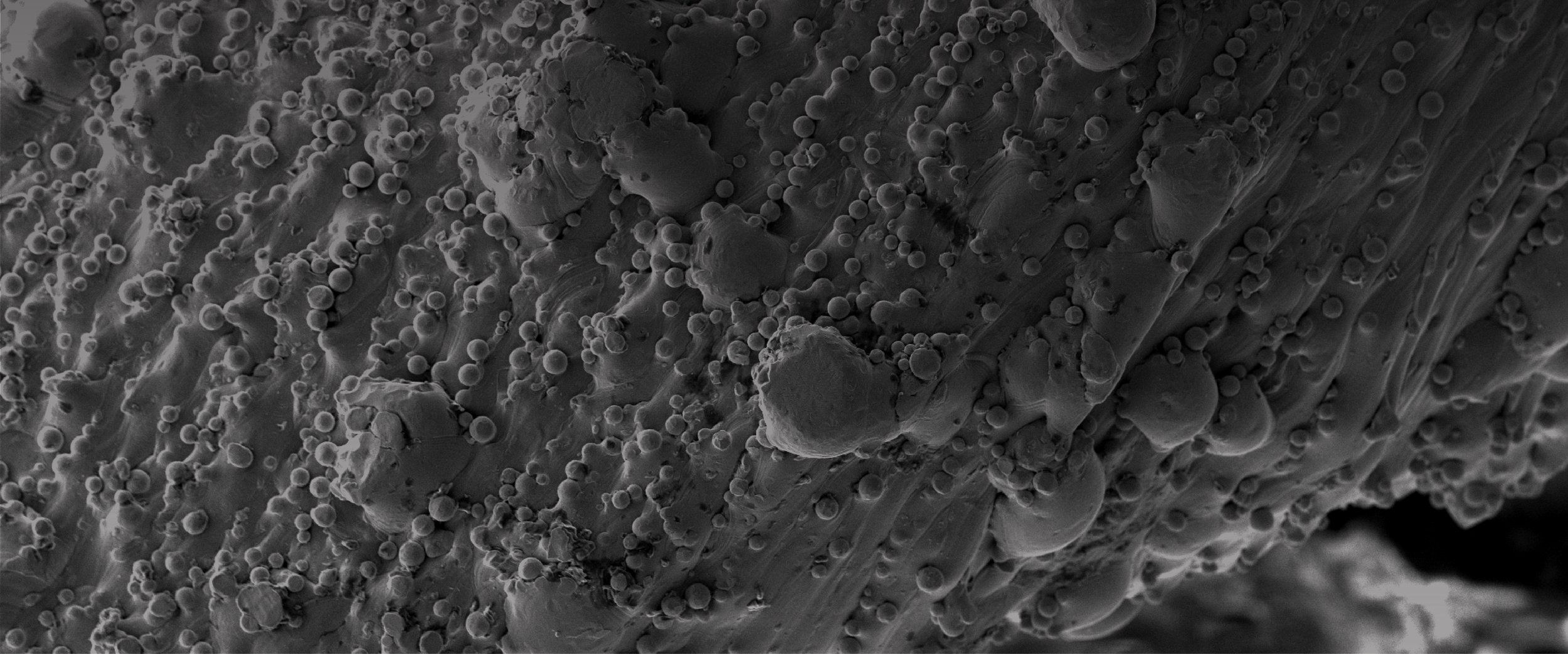
Surface By Design
The Hierarchal surface features are designed to optimize continuous bone on-growth that locks the through fusion to the implant surface. From the literature, it is well understood that a semi-ordered surface with a combination of micro, macro and nano structures drives cellular attachment and gene expression⁴ ⁷.
Provides immediate stability with surface features designed for significant friction to help prevent motion
Encourages bone cell proliferation with roughened titanium surface⁸ ⁹
Promotes “mechanical fusion” bone ingrowth for short-term stability, using trabecular, bone-like surface design, with an average pore diameter of 500µm
190%
Increased expulsion resistance by 190% over traditional PEEK provided by surface technology¹⁰
80%
Up to approximately 80% surface porosity
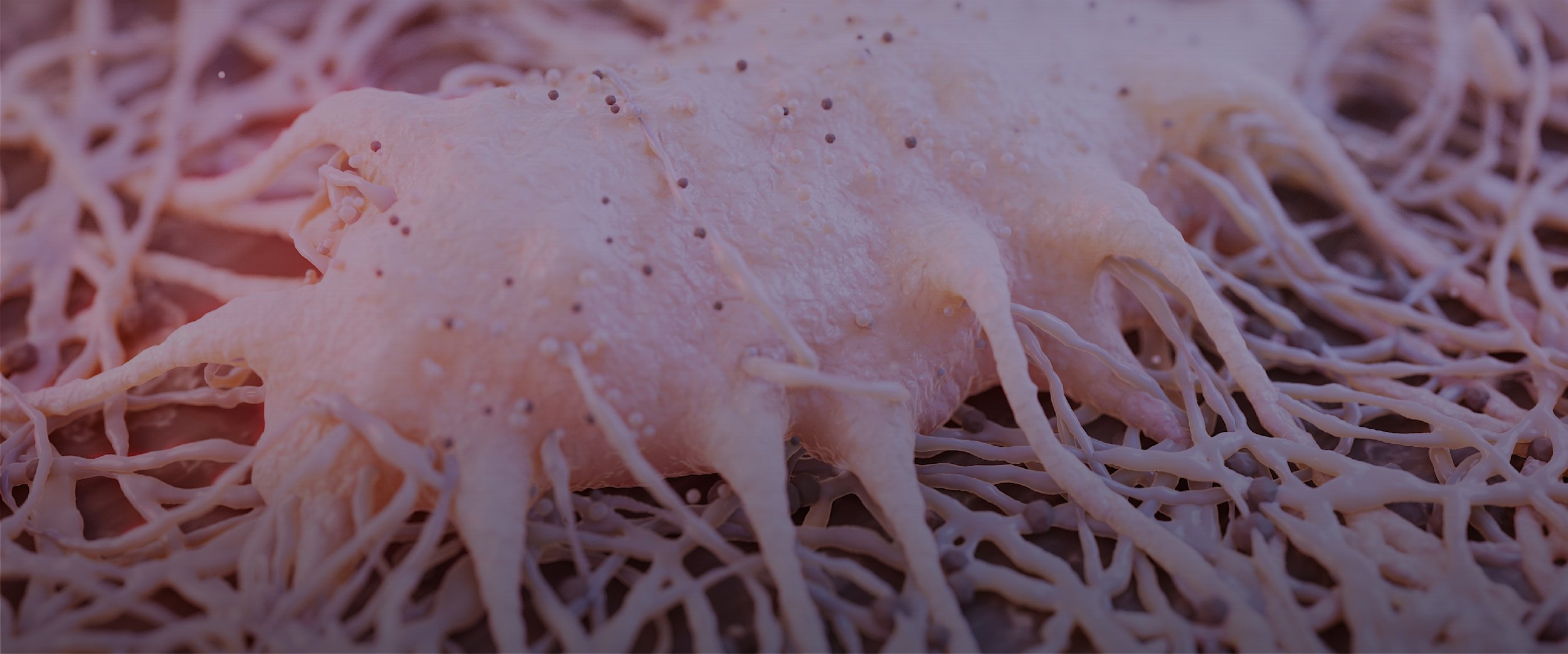
Surrounded By Science
Proven Osteopromotive Surface*
Cellular attachment and filopodia spread are present as early as 7 days. The surfaces with greater microtextures have greater attachment. This activity is the beginning of the mesenchymal stem cell’s commitment towards osteoblastic lineage.
SEM Cell Culture Results: Titanium coupons were 3D printed, cleaned, and sterilized with different surface microtextures, representative of the SPIRA® surface, and cultured with human mesenchymal stem cells at 3 time points (7 days, 14 days, and 28 days).
With current 3D printing technology, optimization of cellular attachment and biological response for osteoblast differentiation and bone formation can be achieved. SPIRA®’s 3D printing technology osteoconductive microtexture allows for early bone apposition and promotes a faster fusion.¹¹
Engineered for better Fusion
A 59-year-old male with long standing neck pain presented when severe, incapacitating radicular arm complaints which far exceeded his existing neck complaints. Imaging revealed advanced degenerative changes at C5-6 and C6-7 with bilateral foraminal stenosis and loss of cervical lordosis (Figure 1).
Figure 1
Figure 2a
Figure 2b
The patient was treated anterior cervical discectomy and fusion with SPIRA®-C and an anterior cervical plate. Computed topography scan at 12 months showed solid fusion with bone through-growth (Figures 2a & 2b).
Access the full version of our SPIRA® Technical Monograph
Open Matrix Cervical Interbody
Integrated Fixation System
Open Matrix ALIF
Open Matrix LLIF
Open Matrix OLIF
Posterior Lumbar Spacer
Oblique Posterior Lumbar Spacer
Open Matrix Corpectomy System
*All data on file
[1] Wolff J. The Law of Bone Remodeling. Berlin Heidelberg New York: Springer, 1986 (translation of the German 1892 edition).
[2] (Internal FEA Report Colorado State) SPA-R194.
[3] Katsuura, Y., Wright-Chisem, J., Wright-Chisem, A. et al. The Importance of Surface Technology in Spinal Fusion. HSS Jrnl 16, 113–116 (2020).
[4] Gittens RA, Olivares-Navarrete R, Schwartz Z, Boyan BD. Implant osseointegration and the role of microroughness and nanostructures: lessons for spine implants. Acta Biomater. 2014 Aug;10(8):3363-71. doi: 10.1016/j.actbio.2014.03.037. Epub 2014 Apr 8. PMID: 24721613; PMCID: PMC4103432.
[5] Kasperkiewicz K, Major R, Sypien A, Kot M, Dyner M, Major Ł, Byrski A, Kopernik M, Lackner JM. Antibacterial Optimization of Highly Deformed Titanium Alloys for Spinal Implants. Molecules. 2021 May 24;26(11):3145. doi: 10.3390/molecules26113145. PMID: 34074062; PMCID: PMC8197332.
[6] Kowalski RJ, Ferrara LA, Benzel EC. Biomechanics of bone fusion. Neurosurg Focus. 2001 April 15.
[7] Landén NX, Li D, Ståhle M. Transition from inflammation to proliferation: a critical step during wound healing. Cell Mol Life Sci. 2016 Oct;73(20):3861-85. Epub 2016 May 14.
[8] Deligianni, D. D., et al. “Effect of surface roughness of the titanium alloy Ti–6Al–4V on human bone marrow cell response and on protein adsorption.” Biomaterials 22.11 (2001): 1241-1251.
[9] Martin, J. Y., et al. “Effect of titanium surface roughness on proliferation, differentiation, and protein synthesis of human osteoblast‐like cells (MG63).” Journal of biomedical materials research 29.3 (1995): 389-401.
[10] SPA-M006 – internal report from 510k.
[11] Paschal G, Paschal P, Avrumova F, Goldman S, Sokunbi G, Lebl D, Cammisa F, Abjornson C. Optimization Analysis of 3D Printed Titanium Surfaces for Mesenchymal Stem Cells. e-Presentation. Society for Minimally Invasive Spine Surgery. (SMISS) 2022 Annual Meeting, Las Vegas, NV. September 2022.